Books and reports by Jan Stafleu
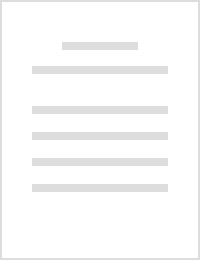
Oceanographic and Marine Cross-Domain Data Management for Sustainable Development, 2017
Van Lancker, V., Francken, F., Kint, L., Terseleer, N., Van den Eynde, D., De Mol, L., De Tré, G.... more Van Lancker, V., Francken, F., Kint, L., Terseleer, N., Van den Eynde, D., De Mol, L., De Tré, G., De Mol, R., Missiaen, T., Hademenos, V., Bakker, M., Busschers, F., Maljers, D., Stafleu, J. and Van Heteren, S., 2017. Building a 4D Voxel-Based Decision Support System for a Sustainable Management of Marine Geological Resources, In: "Oceanographic and Marine Cross-Domain Data Management for Sustainable Development", P. Diviacco, A. Leadbetter and H. Glaves (eds.), IGI Global, doi: 10.4018/978-1-5225-0700-0
For sustainable management of marine geological resources, a geological knowledge base is being built for the Belgian and southern Netherlands part of the North Sea. Voxel models of the subsurface are used for predictions on sand and gravel quantities and qualities, to ensure long-term resource use. The voxels are filled with geological data from boreholes and seismic lines, but other information can be added also. The geology provides boundary conditions needed to run environmental impact models that calculate resource depletion and regeneration under various scenarios of aggregate extraction. Such analyses are important in monitoring progress towards good environmental status, as outlined in the Marine Strategy Framework Directive. By including uncertainty, data products can be generated with confidence limits, which is critical for assessing the significance of changes in the habitat or in any other resource-relevant parameter. All of the information is integrated into a cross-domain, multi-criteria decision support system optimised for user-friendliness and online visualisation.
Final Report. Brussels: Belgian Science Policy 2019 – 75 p. (BRAIN-be - Belgian Research Action through Interdisciplinary Networks), 2019
Van Lancker V, Francken F, Kapel M, Kint L, Terseleer N, Van den Eynde D, Hademenos V, Missiaen T... more Van Lancker V, Francken F, Kapel M, Kint L, Terseleer N, Van den Eynde D, Hademenos V, Missiaen T, De Mol R, De Tré G, Appleton R, van Heteren S, van Maanen PP, Stafleu J, Stam J, Degrendele K, Roche M. Transnational and Integrated Long-term Marine Exploitation Strategies (TILES). Final Report. Brussels: Belgian Science Policy 2019 – 75 p. (BRAIN-be - Belgian Research Action through Interdisciplinary Networks)
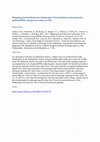
Lauwerier, R.C.G.M., Eerden, M.C., Groenewoudt, B.J., Lascaris, M.A., Rensink, E., Smit, B.I., Speleers, B.P. & Van Doesburg, J. (eds.), Knowledge for Informed Choices – Tools for more effective and efficient selection of valuable archaeology in the Netherlands., 2017
In a geological GIS-data recombination project, a digital map was produced that holds information... more In a geological GIS-data recombination project, a digital map was produced that holds information on the Netherlands’ former coastal and delta plain landscapes in the last 14,000 years: the Holocene and the very end of the Pleistocene. The end product polygon maps are accompanied by a set of palaeoDEMs indicating the ‘attention depth’ for buried land surfaces and aquatic deposits; both for four time slices. This paper provides conceptual background on legend and construction principles of the polygon maps and the palaeoDEMs (‘decisions during the making off’) and a basic overview of the map product (‘landscape structure’, ‘burial depth’, ‘preservation’), visualised for the four time slices of RCE’s Archaeological Knowledge Kit. The text links the coastal plain buried landscape mapping for time slices T0, T1, T2 and T3, to the other Knowledge Kit activities described in this volume, notably that of the Archaeological Landscape map (for time slice T4 in the coastal plain part of The Netherlands, for time slice T0 to T4 in the Pleistocene uplands)

Bordogna G., Carrara P. (eds) Mobile Information Systems Leveraging Volunteered Geographic Information for Earth Observation. Earth Systems Data and Models, vol 4. Springer, Cham, 2018
Geographic decision support systems aim to integrate and process data originating from different ... more Geographic decision support systems aim to integrate and process data originating from different sources and different data providers in order to create suitability models. A suitability model denotes how suitable geographic locations are for a specific purpose on which decision-makers need to make a decision. Particularly in the presence of volunteered information, data quality assessment becomes an important aspect of a decision-making process. Geographic data are commonly prone to incompleteness, imprecision and uncertainty, and this is even more the case with volunteered data. To correctly inform the users, it is essential to communicate not only the suitability degrees highlighted in a suitability model, but also the confidence about these suitability degrees as can be derived from data quality assessment. In this chapter, a novel hierarchical approach for data quality assessment, supporting the computation of associated confidence degrees, is introduced. To illustrate its added value, aspects of the project Transnational and Integrated Long-term marine Exploitation Strategies (TILES) are used. Providing confidence information adds an extra dimension to the decision-making process and leads to more sound decisions.
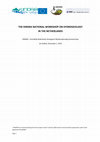
Practical and scientific knowledge related to groundwater research and innovation is scattered am... more Practical and scientific knowledge related to groundwater research and innovation is scattered amongst various actors throughout Europe. KINDRA (Knowledge Inventory for Hydrogeology Research) is developing an inventory of this groundwater knowledge-base, following a new Harmonised Research Classification System (HRC-SYS).
An European Inventory of Groundwater Research (EIGR) is being compiled, including survey results and research activities, projects and programmes, all of which are essential to identify and determine future trends, critical challenges and research gaps. The objective is to improve management and policy development for groundwater resources on a EU level coherently with the Water Framework Directive (WFD) and the Groundwater Directive (GWD).
KINDRA counts on the direct involvement of the European Federation of Geologists (EFG), which will provide the technical expertise of its national members actively cooperating within the project. In case of the Netherlands, the national member is KNGMG.
An important task in the KINDRA project is the organisation of a National Workshop on Hydrogeology in each of the 20 participating countries. KNGMG (Royal Geological and Mining Society of the Netherlands) and NHV (Hydrological Society of the Netherlands) co-organised the Dutch workshop on November 10, 2016. Venue was the office of TNO – Geological Survey of the Netherlands and Deltares in Utrecht.
The workshop was aimed at identifying research gaps in the field of hydrogeology. Research gaps may include missing scientific knowledge about the groundwater system, but also missing information or lack of knowledge of existing information. A second goal of the workshop was to discuss possible solutions to bridge the research gaps.
This report includes the programme of the workshop (Chapter 2), a summary of the presentations (Chapter 3) and a summary of the two discussion round on research gaps (Chapter 4). Chapter 5 lists the participants as well as the professionals who were interested in the workshop but were not able to attend.
Dit rapport beschrijft de hydraulische parameterisering van de holocene eenheden van het GeoTOP m... more Dit rapport beschrijft de hydraulische parameterisering van de holocene eenheden van het GeoTOP modelgebied Zeeland. De nadruk ligt daarbij op het opschalen van in het laboratorium aan monsters gemeten doorlatendheden naar het voxelmodel met voxels van 100 bij 100 bij 0,5 m.
Het rapport beschrijft twee opschalingsmethoden: de "simpele opschaling", bruikbaar voor relatief homogene geologische eenheden, en een nieuw ontwikkelde "complexe opschaling" die geschikt is voor heterogeen samengestelde eenheden. Daarnaast laat het rapport zien hoe GeoTOP met de opgeschaalde waarden wordt gevuld en gebruikt om een kaart van de weerstand (c-waardekaart) van de holocene eenheden te maken. In de discussie is aandacht voor een uitgebreide toets van de gemodelleerde weerstand aan een set van pompproeven in de provincie Zeeland.
This product specification describes the 3D geological subsurface model GeoTOP, version 1.3, prod... more This product specification describes the 3D geological subsurface model GeoTOP, version 1.3, produced by TNO - Geological Survey of the Netherlands. On the basis of this specification the reader cab decide whether or not the GeoTOP model is appropriate for his or her application.
Deze productspecificatie beschrijft het ondergrondmodel GeoTOP, versie 1.3, van TNO – Geologische... more Deze productspecificatie beschrijft het ondergrondmodel GeoTOP, versie 1.3, van TNO – Geologische Dienst Nederland. Op basis van deze specificatie kan de lezer beslissen of, en zo ja hoe, het ondergrondmodel voor zijn of haar toepassing gebruikt kan worden.

The NAM is preparing a new "Winningsplan", to be submitted in 2016. For this new Winningsplan, a ... more The NAM is preparing a new "Winningsplan", to be submitted in 2016. For this new Winningsplan, a new generation of Ground Motion Prediction Equations (GMPEs) will be developed. The overall scope is to reduce uncertainties in the hazard and risk analysis by improvement of input data, such as Groningen-specific data, and better GMPEs. In the current GMPE, only one value for shear wave velocity (Vs) is used for the entire Groningen field (Vs30 = 200 mIs). The shallow subsurface of Groningen, consisting of Holocene and Pleistocene sediments is heterogeneous, resulting in variations of shear wave velocity. It is expected that part of the uncertainties in the seismic hazard and risk analysis can be reduced by including Groningen-specific information and knowledge of the subsurface to improve quantification of the site response caused by earthquakes
Deltares has built a geological model for the Groningen field (+ 5 km buffer) for the purpose of the construction of Vs30 maps and as input for the calculations of site amplification. These results will feed into the new GMPEs. The Geological model for the ~ite response at the Groningen Field (GSG-model) is, among other data sources, based on the beta version of GeoTOP (a 3D geological model of the Netherlands), provided by TNO Geological Survey of the Netherlands. The GSG-model built by Deltares consists of a map defining geological areas and voxel stacks containing stratigraphy and lithological class with depth. Additionally, a state-of-the-art Vs30 map was derived for the Groningen field + 5 km buffer, taking into account Groningen-specific Vs relations and the geology from the GSG-model.
This report describes the method for the construction and the results of version 1 of the GSGmodel, the quality checks performed on the model and recommendations for future versions. When more data becomes available, updates of the GSG-model are anticipated.
TNO Report (in Dutch) about a detailed 3D geological model of the shallow subsurface of a nature ... more TNO Report (in Dutch) about a detailed 3D geological model of the shallow subsurface of a nature reserve in the southern Netherlands.
Deze productspecificatie beschrijft het ondergrondmodel GeoTOP, versie 1.2, van TNO – Geologische... more Deze productspecificatie beschrijft het ondergrondmodel GeoTOP, versie 1.2, van TNO – Geologische Dienst Nederland. Op basis van deze specificatie kan de lezer beslissen of, en zo ja hoe, het ondergrondmodel voor zijn of haar toepassing gebruikt kan worden.

Dit rapport geeft een gedetailleerde beschrijving van GeoTOP: het beschrijft wat GeoTOP is, op we... more Dit rapport geeft een gedetailleerde beschrijving van GeoTOP: het beschrijft wat GeoTOP is, op welke brongegevens het is gebaseerd en hoe het gemaakt wordt. Ook worden de uit GeoTOP afgeleide producten beschreven die (vrijwel) direct toepasbaar zijn bij het analyseren en oplossen van ondergrondvraagstukken.
Het rapport start met een beschrijving van de verschillende onderdelen van het model: geïnterpreteerde boringen, rasters van toppen en basissen van stratigrafische eenheden en voxels met stratigrafische en lithologische kenmerken (hoofdstuk 2). Vervolgens wordt in hoofdstuk 3 een overzicht gegeven van de bij GeoTOP betrokken brongegevens, o.a. boringen, sonderingen en kaartmateriaal. Hoofdstuk 4 beslaat het grootste deel van het rapport en geeft een gedetailleerde beschrijving van het proces waarmee GeoTOP wordt gemaakt. Dit proces bestaat uit 5 werkprocessen: 1) voorbereiden basisgegevens; 2) indelen stratigrafie; 3) modelleren stratigrafie (lagenmodel); 4) modelleren lithoklassen (voxelmodel) en 5) maken afgeleide producten. Het rapport eindigt met een aantal bijlagen die uitleg geven over in GeoTOP gebruikte coderingen en bestandsformaten.

INTRODUCTION
Seismic reflection profiling is the most powerful geophysical method of investiga... more INTRODUCTION
Seismic reflection profiling is the most powerful geophysical method of investigating the upper part of the Earth's crust. Modern two- and three-dimensional seismic surveys provide us with images that look so much like cross-sections through the subsurface, that it is tempting to interpret them as such. However, a seismic section is not the exact equivalent of a geologic cross-section. One of the reasons why such an one-to-one relationship between seismic records and geologic cross-sections does not exist is the limited resolution of seismic waves.
In areas of substantial well control, one-dimensional seismic models of wire line logs can be used to tie high-resolution log readings to the low-resolution seismic information. These models can serve as adequate ground control for identification and interpretation of individual seismic reflections. Understanding seismic sequences and unconformities, however, requires simulation of two-dimensional sections. This thesis presents seismic models of large-scale outcrops to study the seismic resolution of detailed stratigraphic cross-sections.
METHODS
The seismic models in this thesis were constructed using a standard methodology. It consists of the following five steps.
1) Construction of a geologic cross-section that shows the distribution of major lithologies. Large-scale stratal patterns observed on outcrop photos and photomosaics were combined with detailed field measurements. To study resolution problems, the vertical distance over which changes in acoustic properties occur has to be much smaller than the wavelength. 2) Determination of impedance by measuring petrophysical properties of major lithologies or by using published data. 3) Construction of an impedance model, i.e., a cross-section that shows the spatial distribution of velocity and density. The impedance models in this thesis are among the first in which the impedance boundaries mostly coincide with bedding planes. 4) Raytracing to compute a time- or depth section of reflectivity, using own software and commercially available programs. 5) Convolution of the reflectivity section with a source wavelet. Systematic comparisons of seismic models with different frequency contents reveal what frequency is needed to resolve complex stratigraphic features accurately.
CASE STUDIES
Chapters 2 to 7 present case-studies from a variety of areas and stratigraphic intervals (Part I).
Chapter 2 describes the acquisition and processing of shallow seismic surveys on Tertiary outcrops in the Sorbas basin (SE Spain). Seismic models based on outcrop observations, are used in the interpretation of the seismic data.
The seismic models in Chapters 3 to 7 are entirely from carbonate margins or carbonate platform-to-basin transitions. Carbonate platforms are characterized by rapid lateral variations in facies and geometry. The examples show how resolution problems can result from constructive and destructive interference in areas of such rapid lateral facies transitions or other zones of complex sediment geometry.
Examples include the platform-to-basin transition at the Picco di Vallandro and the platform margin of the Sella, both from the Triassic of the Dolomites (Chapters 3 and 4, respectively); the Early Jurassic Djebel Bou Dahar platform and basin-fill geometry (Morocco, Chapter 5); the Early Cretaceous of the Vercors platform (France, Chapter 6); and a mixed carbonate-siliciclastic shelf-margin from the Permian Guadalupe Mountains (New Mexico, USA, Chapter 7).
In some case studies, the seismic models were compared with real seismic records from the same stratigraphic setting (Chapters 2 and 7) or a similar situation (Chapters 5 and 6).
IMPEDANCE DISTRIBUTION
A crucial step in the construction of an outcrop-based seismic model is the conversion of the geologic cross-section into an impedance model showing the distribution of velocity and density (Part II). This can be done by directly measuring the acoustic properties of the rocks or by defining another variable, e.g. gamma-ray or resistivity, that follows the variation of impedance and can serve as a proxy of impedance. Chapter 8 shows how seismic models based on these measurements can be compared with seismic models based on sonic and density logs from boreholes. These comparisons will help to improve methods of converting outcrop data into realistic seismic models.
Erosional topography may be another possible proxy for impedance that is relatively easy to measure in outcrop. The underlying assumption is that resistance against erosion, "erosive hardness", is closely related to impedance, "acoustic hardness". In Chapter 9, photogrammetric techniques were used to establish the relationship between topography and impedance in the Vercors.
CONCLUSIONS
The lithologic detail modeled in the case studies lead to some striking results, particularly the discovery of pseudo-unconformities. Pseudo-unconformities are unconformities in seismics, but correspond to rapid changes of dip and facies in outcrop. In many case studies, lateral amplitude variations did not result from lateral variations in impedance contrasts, but from changes in depositional dip along carbonate platform slopes or from variable bed thickness.
Proxies of velocity, which can be more easily measured than velocity itself, include porosity (if over 10-15%), clay content (if over 10-15%), and bulk density (if both porosity and clay content are less than 10-15%). Another proxy of impedance is erosional relief, expressed as the slope angle.
Papers by Jan Stafleu
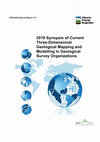
Alberta Energy Regulator / Alberta Geological Survey, AER/AGS Special Report 112, 2019
This paper corresponds to Chapter 17 of Alberta Energy Regulator / Alberta Geological Survey, AER... more This paper corresponds to Chapter 17 of Alberta Energy Regulator / Alberta Geological Survey, AER/AGS Special Report 112, entitled "Synopsis of Current Three-Dimensional Geological Mapping and Modelling in Geological Survey Organizations".
The URL provides a link to the full report. The PDF contains the cover and Chapter 17 only.
Full reference:
Stafleu, J., van der Meulen, M.J., Gunnink, J.L., Maljers, D., Hummelman, J., Busschers, F.S., Schokker, J., Vernes, R.W., Doornenbal, H., den Dulk, M., and ten Veen, J.H., 2019. Systematic 3D subsurface mapping in the Netherlands; Chapter 17 in 2019 Synopsis of Current Three-Dimensional Geological Mapping and Modelling in Geological Survey Organizations, K.E. MacCormack, R.C. Berg, H.A.J. Russell, H. Kessler and L.H. Thorleifson (ed.), Alberta Energy Regulator / Alberta Geological Survey, AER/AGS Special Report 112, p. 179-190.
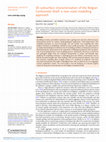
Netherlands Journal of Geosciences, 2019
Hademenos, V., Stafleu, J., Missiaen, T., Kint, L., & Van Lancker, V. (2019). 3D subsurface chara... more Hademenos, V., Stafleu, J., Missiaen, T., Kint, L., & Van Lancker, V. (2019). 3D subsurface characterisation of the Belgian Continental Shelf: A new voxel modelling approach. Netherlands Journal of Geosciences, 98, E1. doi:10.1017/njg.2018.18
Modelling of surface and shallow subsurface data is getting more and more advanced and is demonstrated mostly for onshore (hydro)geological applications. Three-dimensional (3D) modelling techniques are used increasingly, and now include voxel modelling that often employs stochastic or probabilistic methods to assess model uncertainty. This paper presents an adapted methodological workflow for the 3D modelling of offshore sand deposits and aims at demonstrating the improvement of the estimations of lithological properties after incorporation of more geological layers in the modelling process. Importantly, this process is driven by new geological insight from the combined interpretation of seismic and borehole data. Applying 3D modelling techniques is challenging given that offshore environments may be heavily reworked through time, often leading to thin and discontinuous deposits. Since voxel and stochastic modelling allow in-depth analyses of a multitude of properties (and their associated uncertainties) that define a lithological layer, they are ideal for use in an aggregate resource exploitation context. The voxel model is now the backbone of a decision support system for long-term sand extraction on the Belgian Continental Shelf.
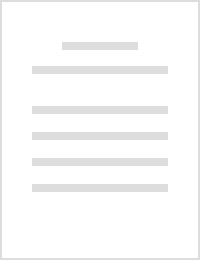
Anthropocene, 2018
Koster, K., Stafleu, J., Cohen, K.M., Stouthamer, E., Busschers, F.S. and Middelkoop, H., 2018. T... more Koster, K., Stafleu, J., Cohen, K.M., Stouthamer, E., Busschers, F.S. and Middelkoop, H., 2018. Three-dimensional distribution of organic matter in coastal-deltaic peat: Implications for subsidence and carbon dioxide emissions by human-induced peat oxidation. Anthropocene, doi: 10.1016/j.ancene.2018.03.001
Human-induced groundwater level lowering in the Holocene coastal-deltaic plain of the Netherlands causes oxidation of peat organic matter, resulting in land subsidence and carbon dioxide (CO2) emissions. Here, a three-dimensional (3D) analysis of the distribution of the remaining peat organic matter is presented, to quantify the potential of this area to further subsidence and CO2 emissions by oxidation. Hereto, we established relations between dry mass ratios of organic matter and sediment in peat formed in different environmental settings. This was combined with a high-resolution 3D geological model of the subsurface of the Netherlands to map the proportions of organic matter, clastic sediment and void space in peat.
The 3D model indicates that c. 15 km3 of Holocene peat is embedded in the coastal-deltaic plain subsurface, of which c. 1.5 km3 consists of organic matter, 0.4 km3 of sediment, and 13.1 km3 of void space. During future human-induced oxidation, this peat has a volumetric loss potential of 14.6 km3, responsible for locally 0.4–6.0 m of subsidence, and a CO2 emission of 2.0 Gton.
The 3D modelling revealed that the amount of peat organic matter varies considerably between regions. Especially the subsurface of urban areas overlying back-barrier peat were identified as hot-spots accommodating the highest quantities of peat organic matter. The peat in agricultural areas contains less organic matter but is more prone to oxidation than peat underlying urban areas, because in the latter settings anthropogenic brought-up soil restricts oxidation. Future mitigation strategies should therefore focus on restricting peat oxidation in the agricultural areas of the coastal-deltaic plain.
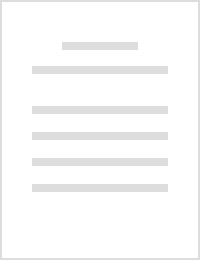
Journal of Coastal Research, 2018
Koster, K., Cohen, K.M., Stafleu, J. and Stouthamer, E., 2018. Using 14C-Dated Peat Beds for Reco... more Koster, K., Cohen, K.M., Stafleu, J. and Stouthamer, E., 2018. Using 14C-Dated Peat Beds for Reconstructing Subsidence by Compression in the Holland Coastal Plain of the Netherlands. Journal of Coastal Research, doi: 10.2112/JCOASTRES-D-17-00093.1
Subsidence in the Holland coastal plain of the Netherlands was reconstructed from the vertical displacement of Holocene peat layers below their reference groundwater levels at the time of peat formation. This quantifies the part of subsidence that is due to compression processes and allows specification of the current state of peat compression in a map. 14C-dating of peat layers found intercalated in the Holocene sequence were used in the reconstruction. This dataset was combined with results from a recent coastal-deltaic plain wide three-dimensional (3D) interpolation of reference palaeo-groundwater levels, at which the intercalated peats are thought to have formed before they were buried, compressed, and vertically displaced. Empiric relations between reconstructed displacement and the thickness of overburden were determined and deployed in a national 3D geological subsurface model to establish a subsidence map with continuous cover of the coastal plain. The resulting maps show compressed peat layers under urbanized areas with 1 to 8 m of natural and anthropogenic overburden have subsided 1 to 5 m below the original level of formation. In the agricultural area of the coastal plain, where overburden is merely decimetres thick, consisting of fluvial flood- and sea-ingression deposits, peat generally experienced less than 1 m subsidence. The reference-level reconstruction method is deployable over large coastal plain areas to reconstruct subsidence caused by postdepositional vertical displacement of intercalated peat layers. It could therefore serve as an alternative approach for methods based on soil mechanics, which require input often not available for coastal plains on regional scales.

Netherlands Journal of Geosciences, 2018
Koster, K., Stafleu, J. & Stouthamer, E., 2018. Differential subsidence in the urbanized coastal-... more Koster, K., Stafleu, J. & Stouthamer, E., 2018. Differential subsidence in the urbanized coastal-deltaic plain of the Netherlands. Netherlands Journal of Geosciences, doi: 10.1017/njg.2018.11
The urbanised peat-rich coastal-deltaic plain of the Netherlands is severely subsiding due to human-induced phreatic groundwater level lowering, as this causes peat layers to compress and oxidise. To determine the potential susceptibility of this area to future subsidence by peat compression and oxidation, the effects of lowering present-day phreatic groundwater levels were quantitatively evaluated using a subsidence model. Input were a 3D geological subsurface voxel-model, modelled phreatic groundwater levels, and functions for peat compression and oxidation. Phreatic groundwater levels were lowered by 0.25 and 0.5 m, and the resulting peat compression and oxidation over periods of 15 and 30 years were determined. The model area comprised the major cities Amsterdam and Rotterdam, and their surrounding agricultural lands. The results revealed that for these scenarios agricultural areas may subside between 0.3 and 0.8 m; potential subsidence in Amsterdam and Rotterdam is considerably lower, less than 0.4 m. This is due to the presence of several metres thick anthropogenic brought-up soils overlying the peat below the urban areas, which has already compressed the peat to a depth below groundwater level, and thus minimises further compression and oxidation. In agricultural areas peat is often situated near the surface, and is therefore highly compressible and prone to oxidation. The averaged subsidence rates for the scenarios range between 7 and 13 mm a −1 , which is corresponds to present-day rates of subsidence in the peat areas of the Netherlands. These results contrast with the trend of coastal-deltaic subsidence in other deltas, with cities subsiding faster than agricultural areas. This difference is explained by the driver of subsidence: in other deltas, subsidence of urban areas is mainly due to deep aquifer extraction, whereas in the Netherlands subsidence is due to phreatic groundwater level lowering.
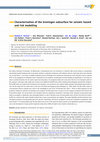
Netherlands Journal of Geosciences, 2017
Kruiver, P.P., Wiersma, A., Kloosterman, F.H., De Lange, G., Korff, M., Stafleu, J., Busschers, F... more Kruiver, P.P., Wiersma, A., Kloosterman, F.H., De Lange, G., Korff, M., Stafleu, J., Busschers, F.S., Harting, R., Gunnink, J.L., Green, R.A., Van Elk, J. and Doornhof, D., 2017. Characterisation of the Groningen subsurface for seismic hazard and risk modelling. Netherlands Journal of Geosciences, 96: 215-233, doi:10.1017/njg.2017.11
The shallow subsurface of Groningen, the Netherlands, is heterogeneous due to its formation in a Holocene tidal coastal setting on a periglacially and glacially inherited landscape with strong lateral variation in subsurface architecture. Soft sediments with low, small-strain shear wave velocities (V S30 around 200 m s −1) are known to amplify earthquake motions. Knowledge of the architecture and properties of the subsurface and the combined effect on the propagation of earthquake waves is imperative for the prediction of geohazards of ground shaking and liquefaction at the surface. In order to provide information for the seismic hazard and risk analysis, two geological models were constructed. The first is the 'Geological model for Site response in Groningen' (GSG model) and is based on the detailed 3D GeoTOP voxel model containing lithostratigraphy and lithoclass attributes. The GeoTOP model was combined with information from boreholes, cone penetration tests, regional digital geological and geohydrological models to cover the full range from the surface down to the base of the North Sea Supergroup (base Paleogene) at ∼800 m depth. The GSG model consists of a microzonation based on geology and a stack of soil stratigraphy for each of the 140,000 grid cells (100 m × 100 m) to which properties (V S and parameters relevant for nonlinear soil behaviour) were assigned. The GSG model serves as input to the site response calculations that feed into the Ground Motion Model. The second model is the 'Geological model for Liquefaction sensitivity in Groningen' (GLG). Generally, loosely packed sands might be susceptible to liquefaction upon earthquake shaking. In order to delineate zones of loosely packed sand in the first 40 m below the surface, GeoTOP was combined with relative densities inferred from a large cone penetration test database. The marine Naaldwijk and Eem Formations have the highest proportion of loosely packed sand (31% and 38%, respectively) and thus are considered to be the most vulnerable to liquefaction; other units contain 5–17% loosely packed sand. The GLG model serves as one of the inputs for further research on the liquefaction potential in Groningen, such as the development of region-specific magnitude scaling factors (MSF) and depth–stress reduction relationships (r d).
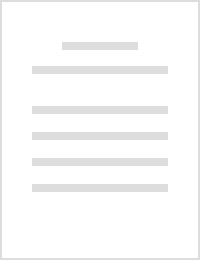
Bull. Earthquake Eng., 2017
Kruiver, P.P., Van Dedem, E., Romijn, R., De Lange, G., Korff, M., Stafleu, J., Gunnink, J.L., Ro... more Kruiver, P.P., Van Dedem, E., Romijn, R., De Lange, G., Korff, M., Stafleu, J., Gunnink, J.L., Rodriguez-Marek, A., Bommer, J.J., Van Elk, J., Doornhof, D., 2017. An integrated shear-wave velocity model for the Groningen gas field, The Netherlands. Bull. Earthquake Eng. 15: 3555-3580, doi: 10.1007/s10518-017-0105-y
A regional shear-wave velocity (VS) model has been developed for the Groningen gas field in the Netherlands as the basis for seismic microzonation of an area of more than 1000 km2. The VS model, extending to a depth of almost 1 km, is an essential input to the modelling of hazard and risk due to induced earthquakes in the region. The detailed VS profiles are constructed from a novel combination of three data sets covering different, partially overlapping depth ranges. The uppermost 50 m of the VS profiles are obtained from a high-resolution geological model with representative VS values assigned to the sediments. Field measurements of VS were used to derive representative VS values for the different types of sediments. The profiles from 50 to 120 m are obtained from inversion of surface waves recorded (as noise) during deep seismic reflection profiling of the gas reservoir. The deepest part of the profiles is obtained from sonic logging and VP–VS relationships based on measurements in deep boreholes. Criteria were established for the splicing of the three portions to generate continuous models over the entire depth range for use in site response calculations, for which an elastic half-space is assumed to exist below a clear stratigraphic boundary and impedance contrast encountered at about 800 m depth. In order to facilitate fully probabilistic site response analyses, a scheme for the randomisation of the VS profiles is implemented.
Uploads
Books and reports by Jan Stafleu
For sustainable management of marine geological resources, a geological knowledge base is being built for the Belgian and southern Netherlands part of the North Sea. Voxel models of the subsurface are used for predictions on sand and gravel quantities and qualities, to ensure long-term resource use. The voxels are filled with geological data from boreholes and seismic lines, but other information can be added also. The geology provides boundary conditions needed to run environmental impact models that calculate resource depletion and regeneration under various scenarios of aggregate extraction. Such analyses are important in monitoring progress towards good environmental status, as outlined in the Marine Strategy Framework Directive. By including uncertainty, data products can be generated with confidence limits, which is critical for assessing the significance of changes in the habitat or in any other resource-relevant parameter. All of the information is integrated into a cross-domain, multi-criteria decision support system optimised for user-friendliness and online visualisation.
An European Inventory of Groundwater Research (EIGR) is being compiled, including survey results and research activities, projects and programmes, all of which are essential to identify and determine future trends, critical challenges and research gaps. The objective is to improve management and policy development for groundwater resources on a EU level coherently with the Water Framework Directive (WFD) and the Groundwater Directive (GWD).
KINDRA counts on the direct involvement of the European Federation of Geologists (EFG), which will provide the technical expertise of its national members actively cooperating within the project. In case of the Netherlands, the national member is KNGMG.
An important task in the KINDRA project is the organisation of a National Workshop on Hydrogeology in each of the 20 participating countries. KNGMG (Royal Geological and Mining Society of the Netherlands) and NHV (Hydrological Society of the Netherlands) co-organised the Dutch workshop on November 10, 2016. Venue was the office of TNO – Geological Survey of the Netherlands and Deltares in Utrecht.
The workshop was aimed at identifying research gaps in the field of hydrogeology. Research gaps may include missing scientific knowledge about the groundwater system, but also missing information or lack of knowledge of existing information. A second goal of the workshop was to discuss possible solutions to bridge the research gaps.
This report includes the programme of the workshop (Chapter 2), a summary of the presentations (Chapter 3) and a summary of the two discussion round on research gaps (Chapter 4). Chapter 5 lists the participants as well as the professionals who were interested in the workshop but were not able to attend.
Het rapport beschrijft twee opschalingsmethoden: de "simpele opschaling", bruikbaar voor relatief homogene geologische eenheden, en een nieuw ontwikkelde "complexe opschaling" die geschikt is voor heterogeen samengestelde eenheden. Daarnaast laat het rapport zien hoe GeoTOP met de opgeschaalde waarden wordt gevuld en gebruikt om een kaart van de weerstand (c-waardekaart) van de holocene eenheden te maken. In de discussie is aandacht voor een uitgebreide toets van de gemodelleerde weerstand aan een set van pompproeven in de provincie Zeeland.
Deltares has built a geological model for the Groningen field (+ 5 km buffer) for the purpose of the construction of Vs30 maps and as input for the calculations of site amplification. These results will feed into the new GMPEs. The Geological model for the ~ite response at the Groningen Field (GSG-model) is, among other data sources, based on the beta version of GeoTOP (a 3D geological model of the Netherlands), provided by TNO Geological Survey of the Netherlands. The GSG-model built by Deltares consists of a map defining geological areas and voxel stacks containing stratigraphy and lithological class with depth. Additionally, a state-of-the-art Vs30 map was derived for the Groningen field + 5 km buffer, taking into account Groningen-specific Vs relations and the geology from the GSG-model.
This report describes the method for the construction and the results of version 1 of the GSGmodel, the quality checks performed on the model and recommendations for future versions. When more data becomes available, updates of the GSG-model are anticipated.
Het rapport start met een beschrijving van de verschillende onderdelen van het model: geïnterpreteerde boringen, rasters van toppen en basissen van stratigrafische eenheden en voxels met stratigrafische en lithologische kenmerken (hoofdstuk 2). Vervolgens wordt in hoofdstuk 3 een overzicht gegeven van de bij GeoTOP betrokken brongegevens, o.a. boringen, sonderingen en kaartmateriaal. Hoofdstuk 4 beslaat het grootste deel van het rapport en geeft een gedetailleerde beschrijving van het proces waarmee GeoTOP wordt gemaakt. Dit proces bestaat uit 5 werkprocessen: 1) voorbereiden basisgegevens; 2) indelen stratigrafie; 3) modelleren stratigrafie (lagenmodel); 4) modelleren lithoklassen (voxelmodel) en 5) maken afgeleide producten. Het rapport eindigt met een aantal bijlagen die uitleg geven over in GeoTOP gebruikte coderingen en bestandsformaten.
Seismic reflection profiling is the most powerful geophysical method of investigating the upper part of the Earth's crust. Modern two- and three-dimensional seismic surveys provide us with images that look so much like cross-sections through the subsurface, that it is tempting to interpret them as such. However, a seismic section is not the exact equivalent of a geologic cross-section. One of the reasons why such an one-to-one relationship between seismic records and geologic cross-sections does not exist is the limited resolution of seismic waves.
In areas of substantial well control, one-dimensional seismic models of wire line logs can be used to tie high-resolution log readings to the low-resolution seismic information. These models can serve as adequate ground control for identification and interpretation of individual seismic reflections. Understanding seismic sequences and unconformities, however, requires simulation of two-dimensional sections. This thesis presents seismic models of large-scale outcrops to study the seismic resolution of detailed stratigraphic cross-sections.
METHODS
The seismic models in this thesis were constructed using a standard methodology. It consists of the following five steps.
1) Construction of a geologic cross-section that shows the distribution of major lithologies. Large-scale stratal patterns observed on outcrop photos and photomosaics were combined with detailed field measurements. To study resolution problems, the vertical distance over which changes in acoustic properties occur has to be much smaller than the wavelength. 2) Determination of impedance by measuring petrophysical properties of major lithologies or by using published data. 3) Construction of an impedance model, i.e., a cross-section that shows the spatial distribution of velocity and density. The impedance models in this thesis are among the first in which the impedance boundaries mostly coincide with bedding planes. 4) Raytracing to compute a time- or depth section of reflectivity, using own software and commercially available programs. 5) Convolution of the reflectivity section with a source wavelet. Systematic comparisons of seismic models with different frequency contents reveal what frequency is needed to resolve complex stratigraphic features accurately.
CASE STUDIES
Chapters 2 to 7 present case-studies from a variety of areas and stratigraphic intervals (Part I).
Chapter 2 describes the acquisition and processing of shallow seismic surveys on Tertiary outcrops in the Sorbas basin (SE Spain). Seismic models based on outcrop observations, are used in the interpretation of the seismic data.
The seismic models in Chapters 3 to 7 are entirely from carbonate margins or carbonate platform-to-basin transitions. Carbonate platforms are characterized by rapid lateral variations in facies and geometry. The examples show how resolution problems can result from constructive and destructive interference in areas of such rapid lateral facies transitions or other zones of complex sediment geometry.
Examples include the platform-to-basin transition at the Picco di Vallandro and the platform margin of the Sella, both from the Triassic of the Dolomites (Chapters 3 and 4, respectively); the Early Jurassic Djebel Bou Dahar platform and basin-fill geometry (Morocco, Chapter 5); the Early Cretaceous of the Vercors platform (France, Chapter 6); and a mixed carbonate-siliciclastic shelf-margin from the Permian Guadalupe Mountains (New Mexico, USA, Chapter 7).
In some case studies, the seismic models were compared with real seismic records from the same stratigraphic setting (Chapters 2 and 7) or a similar situation (Chapters 5 and 6).
IMPEDANCE DISTRIBUTION
A crucial step in the construction of an outcrop-based seismic model is the conversion of the geologic cross-section into an impedance model showing the distribution of velocity and density (Part II). This can be done by directly measuring the acoustic properties of the rocks or by defining another variable, e.g. gamma-ray or resistivity, that follows the variation of impedance and can serve as a proxy of impedance. Chapter 8 shows how seismic models based on these measurements can be compared with seismic models based on sonic and density logs from boreholes. These comparisons will help to improve methods of converting outcrop data into realistic seismic models.
Erosional topography may be another possible proxy for impedance that is relatively easy to measure in outcrop. The underlying assumption is that resistance against erosion, "erosive hardness", is closely related to impedance, "acoustic hardness". In Chapter 9, photogrammetric techniques were used to establish the relationship between topography and impedance in the Vercors.
CONCLUSIONS
The lithologic detail modeled in the case studies lead to some striking results, particularly the discovery of pseudo-unconformities. Pseudo-unconformities are unconformities in seismics, but correspond to rapid changes of dip and facies in outcrop. In many case studies, lateral amplitude variations did not result from lateral variations in impedance contrasts, but from changes in depositional dip along carbonate platform slopes or from variable bed thickness.
Proxies of velocity, which can be more easily measured than velocity itself, include porosity (if over 10-15%), clay content (if over 10-15%), and bulk density (if both porosity and clay content are less than 10-15%). Another proxy of impedance is erosional relief, expressed as the slope angle.
Papers by Jan Stafleu
The URL provides a link to the full report. The PDF contains the cover and Chapter 17 only.
Full reference:
Stafleu, J., van der Meulen, M.J., Gunnink, J.L., Maljers, D., Hummelman, J., Busschers, F.S., Schokker, J., Vernes, R.W., Doornenbal, H., den Dulk, M., and ten Veen, J.H., 2019. Systematic 3D subsurface mapping in the Netherlands; Chapter 17 in 2019 Synopsis of Current Three-Dimensional Geological Mapping and Modelling in Geological Survey Organizations, K.E. MacCormack, R.C. Berg, H.A.J. Russell, H. Kessler and L.H. Thorleifson (ed.), Alberta Energy Regulator / Alberta Geological Survey, AER/AGS Special Report 112, p. 179-190.
Modelling of surface and shallow subsurface data is getting more and more advanced and is demonstrated mostly for onshore (hydro)geological applications. Three-dimensional (3D) modelling techniques are used increasingly, and now include voxel modelling that often employs stochastic or probabilistic methods to assess model uncertainty. This paper presents an adapted methodological workflow for the 3D modelling of offshore sand deposits and aims at demonstrating the improvement of the estimations of lithological properties after incorporation of more geological layers in the modelling process. Importantly, this process is driven by new geological insight from the combined interpretation of seismic and borehole data. Applying 3D modelling techniques is challenging given that offshore environments may be heavily reworked through time, often leading to thin and discontinuous deposits. Since voxel and stochastic modelling allow in-depth analyses of a multitude of properties (and their associated uncertainties) that define a lithological layer, they are ideal for use in an aggregate resource exploitation context. The voxel model is now the backbone of a decision support system for long-term sand extraction on the Belgian Continental Shelf.
Human-induced groundwater level lowering in the Holocene coastal-deltaic plain of the Netherlands causes oxidation of peat organic matter, resulting in land subsidence and carbon dioxide (CO2) emissions. Here, a three-dimensional (3D) analysis of the distribution of the remaining peat organic matter is presented, to quantify the potential of this area to further subsidence and CO2 emissions by oxidation. Hereto, we established relations between dry mass ratios of organic matter and sediment in peat formed in different environmental settings. This was combined with a high-resolution 3D geological model of the subsurface of the Netherlands to map the proportions of organic matter, clastic sediment and void space in peat.
The 3D model indicates that c. 15 km3 of Holocene peat is embedded in the coastal-deltaic plain subsurface, of which c. 1.5 km3 consists of organic matter, 0.4 km3 of sediment, and 13.1 km3 of void space. During future human-induced oxidation, this peat has a volumetric loss potential of 14.6 km3, responsible for locally 0.4–6.0 m of subsidence, and a CO2 emission of 2.0 Gton.
The 3D modelling revealed that the amount of peat organic matter varies considerably between regions. Especially the subsurface of urban areas overlying back-barrier peat were identified as hot-spots accommodating the highest quantities of peat organic matter. The peat in agricultural areas contains less organic matter but is more prone to oxidation than peat underlying urban areas, because in the latter settings anthropogenic brought-up soil restricts oxidation. Future mitigation strategies should therefore focus on restricting peat oxidation in the agricultural areas of the coastal-deltaic plain.
Subsidence in the Holland coastal plain of the Netherlands was reconstructed from the vertical displacement of Holocene peat layers below their reference groundwater levels at the time of peat formation. This quantifies the part of subsidence that is due to compression processes and allows specification of the current state of peat compression in a map. 14C-dating of peat layers found intercalated in the Holocene sequence were used in the reconstruction. This dataset was combined with results from a recent coastal-deltaic plain wide three-dimensional (3D) interpolation of reference palaeo-groundwater levels, at which the intercalated peats are thought to have formed before they were buried, compressed, and vertically displaced. Empiric relations between reconstructed displacement and the thickness of overburden were determined and deployed in a national 3D geological subsurface model to establish a subsidence map with continuous cover of the coastal plain. The resulting maps show compressed peat layers under urbanized areas with 1 to 8 m of natural and anthropogenic overburden have subsided 1 to 5 m below the original level of formation. In the agricultural area of the coastal plain, where overburden is merely decimetres thick, consisting of fluvial flood- and sea-ingression deposits, peat generally experienced less than 1 m subsidence. The reference-level reconstruction method is deployable over large coastal plain areas to reconstruct subsidence caused by postdepositional vertical displacement of intercalated peat layers. It could therefore serve as an alternative approach for methods based on soil mechanics, which require input often not available for coastal plains on regional scales.
The urbanised peat-rich coastal-deltaic plain of the Netherlands is severely subsiding due to human-induced phreatic groundwater level lowering, as this causes peat layers to compress and oxidise. To determine the potential susceptibility of this area to future subsidence by peat compression and oxidation, the effects of lowering present-day phreatic groundwater levels were quantitatively evaluated using a subsidence model. Input were a 3D geological subsurface voxel-model, modelled phreatic groundwater levels, and functions for peat compression and oxidation. Phreatic groundwater levels were lowered by 0.25 and 0.5 m, and the resulting peat compression and oxidation over periods of 15 and 30 years were determined. The model area comprised the major cities Amsterdam and Rotterdam, and their surrounding agricultural lands. The results revealed that for these scenarios agricultural areas may subside between 0.3 and 0.8 m; potential subsidence in Amsterdam and Rotterdam is considerably lower, less than 0.4 m. This is due to the presence of several metres thick anthropogenic brought-up soils overlying the peat below the urban areas, which has already compressed the peat to a depth below groundwater level, and thus minimises further compression and oxidation. In agricultural areas peat is often situated near the surface, and is therefore highly compressible and prone to oxidation. The averaged subsidence rates for the scenarios range between 7 and 13 mm a −1 , which is corresponds to present-day rates of subsidence in the peat areas of the Netherlands. These results contrast with the trend of coastal-deltaic subsidence in other deltas, with cities subsiding faster than agricultural areas. This difference is explained by the driver of subsidence: in other deltas, subsidence of urban areas is mainly due to deep aquifer extraction, whereas in the Netherlands subsidence is due to phreatic groundwater level lowering.
The shallow subsurface of Groningen, the Netherlands, is heterogeneous due to its formation in a Holocene tidal coastal setting on a periglacially and glacially inherited landscape with strong lateral variation in subsurface architecture. Soft sediments with low, small-strain shear wave velocities (V S30 around 200 m s −1) are known to amplify earthquake motions. Knowledge of the architecture and properties of the subsurface and the combined effect on the propagation of earthquake waves is imperative for the prediction of geohazards of ground shaking and liquefaction at the surface. In order to provide information for the seismic hazard and risk analysis, two geological models were constructed. The first is the 'Geological model for Site response in Groningen' (GSG model) and is based on the detailed 3D GeoTOP voxel model containing lithostratigraphy and lithoclass attributes. The GeoTOP model was combined with information from boreholes, cone penetration tests, regional digital geological and geohydrological models to cover the full range from the surface down to the base of the North Sea Supergroup (base Paleogene) at ∼800 m depth. The GSG model consists of a microzonation based on geology and a stack of soil stratigraphy for each of the 140,000 grid cells (100 m × 100 m) to which properties (V S and parameters relevant for nonlinear soil behaviour) were assigned. The GSG model serves as input to the site response calculations that feed into the Ground Motion Model. The second model is the 'Geological model for Liquefaction sensitivity in Groningen' (GLG). Generally, loosely packed sands might be susceptible to liquefaction upon earthquake shaking. In order to delineate zones of loosely packed sand in the first 40 m below the surface, GeoTOP was combined with relative densities inferred from a large cone penetration test database. The marine Naaldwijk and Eem Formations have the highest proportion of loosely packed sand (31% and 38%, respectively) and thus are considered to be the most vulnerable to liquefaction; other units contain 5–17% loosely packed sand. The GLG model serves as one of the inputs for further research on the liquefaction potential in Groningen, such as the development of region-specific magnitude scaling factors (MSF) and depth–stress reduction relationships (r d).
A regional shear-wave velocity (VS) model has been developed for the Groningen gas field in the Netherlands as the basis for seismic microzonation of an area of more than 1000 km2. The VS model, extending to a depth of almost 1 km, is an essential input to the modelling of hazard and risk due to induced earthquakes in the region. The detailed VS profiles are constructed from a novel combination of three data sets covering different, partially overlapping depth ranges. The uppermost 50 m of the VS profiles are obtained from a high-resolution geological model with representative VS values assigned to the sediments. Field measurements of VS were used to derive representative VS values for the different types of sediments. The profiles from 50 to 120 m are obtained from inversion of surface waves recorded (as noise) during deep seismic reflection profiling of the gas reservoir. The deepest part of the profiles is obtained from sonic logging and VP–VS relationships based on measurements in deep boreholes. Criteria were established for the splicing of the three portions to generate continuous models over the entire depth range for use in site response calculations, for which an elastic half-space is assumed to exist below a clear stratigraphic boundary and impedance contrast encountered at about 800 m depth. In order to facilitate fully probabilistic site response analyses, a scheme for the randomisation of the VS profiles is implemented.
For sustainable management of marine geological resources, a geological knowledge base is being built for the Belgian and southern Netherlands part of the North Sea. Voxel models of the subsurface are used for predictions on sand and gravel quantities and qualities, to ensure long-term resource use. The voxels are filled with geological data from boreholes and seismic lines, but other information can be added also. The geology provides boundary conditions needed to run environmental impact models that calculate resource depletion and regeneration under various scenarios of aggregate extraction. Such analyses are important in monitoring progress towards good environmental status, as outlined in the Marine Strategy Framework Directive. By including uncertainty, data products can be generated with confidence limits, which is critical for assessing the significance of changes in the habitat or in any other resource-relevant parameter. All of the information is integrated into a cross-domain, multi-criteria decision support system optimised for user-friendliness and online visualisation.
An European Inventory of Groundwater Research (EIGR) is being compiled, including survey results and research activities, projects and programmes, all of which are essential to identify and determine future trends, critical challenges and research gaps. The objective is to improve management and policy development for groundwater resources on a EU level coherently with the Water Framework Directive (WFD) and the Groundwater Directive (GWD).
KINDRA counts on the direct involvement of the European Federation of Geologists (EFG), which will provide the technical expertise of its national members actively cooperating within the project. In case of the Netherlands, the national member is KNGMG.
An important task in the KINDRA project is the organisation of a National Workshop on Hydrogeology in each of the 20 participating countries. KNGMG (Royal Geological and Mining Society of the Netherlands) and NHV (Hydrological Society of the Netherlands) co-organised the Dutch workshop on November 10, 2016. Venue was the office of TNO – Geological Survey of the Netherlands and Deltares in Utrecht.
The workshop was aimed at identifying research gaps in the field of hydrogeology. Research gaps may include missing scientific knowledge about the groundwater system, but also missing information or lack of knowledge of existing information. A second goal of the workshop was to discuss possible solutions to bridge the research gaps.
This report includes the programme of the workshop (Chapter 2), a summary of the presentations (Chapter 3) and a summary of the two discussion round on research gaps (Chapter 4). Chapter 5 lists the participants as well as the professionals who were interested in the workshop but were not able to attend.
Het rapport beschrijft twee opschalingsmethoden: de "simpele opschaling", bruikbaar voor relatief homogene geologische eenheden, en een nieuw ontwikkelde "complexe opschaling" die geschikt is voor heterogeen samengestelde eenheden. Daarnaast laat het rapport zien hoe GeoTOP met de opgeschaalde waarden wordt gevuld en gebruikt om een kaart van de weerstand (c-waardekaart) van de holocene eenheden te maken. In de discussie is aandacht voor een uitgebreide toets van de gemodelleerde weerstand aan een set van pompproeven in de provincie Zeeland.
Deltares has built a geological model for the Groningen field (+ 5 km buffer) for the purpose of the construction of Vs30 maps and as input for the calculations of site amplification. These results will feed into the new GMPEs. The Geological model for the ~ite response at the Groningen Field (GSG-model) is, among other data sources, based on the beta version of GeoTOP (a 3D geological model of the Netherlands), provided by TNO Geological Survey of the Netherlands. The GSG-model built by Deltares consists of a map defining geological areas and voxel stacks containing stratigraphy and lithological class with depth. Additionally, a state-of-the-art Vs30 map was derived for the Groningen field + 5 km buffer, taking into account Groningen-specific Vs relations and the geology from the GSG-model.
This report describes the method for the construction and the results of version 1 of the GSGmodel, the quality checks performed on the model and recommendations for future versions. When more data becomes available, updates of the GSG-model are anticipated.
Het rapport start met een beschrijving van de verschillende onderdelen van het model: geïnterpreteerde boringen, rasters van toppen en basissen van stratigrafische eenheden en voxels met stratigrafische en lithologische kenmerken (hoofdstuk 2). Vervolgens wordt in hoofdstuk 3 een overzicht gegeven van de bij GeoTOP betrokken brongegevens, o.a. boringen, sonderingen en kaartmateriaal. Hoofdstuk 4 beslaat het grootste deel van het rapport en geeft een gedetailleerde beschrijving van het proces waarmee GeoTOP wordt gemaakt. Dit proces bestaat uit 5 werkprocessen: 1) voorbereiden basisgegevens; 2) indelen stratigrafie; 3) modelleren stratigrafie (lagenmodel); 4) modelleren lithoklassen (voxelmodel) en 5) maken afgeleide producten. Het rapport eindigt met een aantal bijlagen die uitleg geven over in GeoTOP gebruikte coderingen en bestandsformaten.
Seismic reflection profiling is the most powerful geophysical method of investigating the upper part of the Earth's crust. Modern two- and three-dimensional seismic surveys provide us with images that look so much like cross-sections through the subsurface, that it is tempting to interpret them as such. However, a seismic section is not the exact equivalent of a geologic cross-section. One of the reasons why such an one-to-one relationship between seismic records and geologic cross-sections does not exist is the limited resolution of seismic waves.
In areas of substantial well control, one-dimensional seismic models of wire line logs can be used to tie high-resolution log readings to the low-resolution seismic information. These models can serve as adequate ground control for identification and interpretation of individual seismic reflections. Understanding seismic sequences and unconformities, however, requires simulation of two-dimensional sections. This thesis presents seismic models of large-scale outcrops to study the seismic resolution of detailed stratigraphic cross-sections.
METHODS
The seismic models in this thesis were constructed using a standard methodology. It consists of the following five steps.
1) Construction of a geologic cross-section that shows the distribution of major lithologies. Large-scale stratal patterns observed on outcrop photos and photomosaics were combined with detailed field measurements. To study resolution problems, the vertical distance over which changes in acoustic properties occur has to be much smaller than the wavelength. 2) Determination of impedance by measuring petrophysical properties of major lithologies or by using published data. 3) Construction of an impedance model, i.e., a cross-section that shows the spatial distribution of velocity and density. The impedance models in this thesis are among the first in which the impedance boundaries mostly coincide with bedding planes. 4) Raytracing to compute a time- or depth section of reflectivity, using own software and commercially available programs. 5) Convolution of the reflectivity section with a source wavelet. Systematic comparisons of seismic models with different frequency contents reveal what frequency is needed to resolve complex stratigraphic features accurately.
CASE STUDIES
Chapters 2 to 7 present case-studies from a variety of areas and stratigraphic intervals (Part I).
Chapter 2 describes the acquisition and processing of shallow seismic surveys on Tertiary outcrops in the Sorbas basin (SE Spain). Seismic models based on outcrop observations, are used in the interpretation of the seismic data.
The seismic models in Chapters 3 to 7 are entirely from carbonate margins or carbonate platform-to-basin transitions. Carbonate platforms are characterized by rapid lateral variations in facies and geometry. The examples show how resolution problems can result from constructive and destructive interference in areas of such rapid lateral facies transitions or other zones of complex sediment geometry.
Examples include the platform-to-basin transition at the Picco di Vallandro and the platform margin of the Sella, both from the Triassic of the Dolomites (Chapters 3 and 4, respectively); the Early Jurassic Djebel Bou Dahar platform and basin-fill geometry (Morocco, Chapter 5); the Early Cretaceous of the Vercors platform (France, Chapter 6); and a mixed carbonate-siliciclastic shelf-margin from the Permian Guadalupe Mountains (New Mexico, USA, Chapter 7).
In some case studies, the seismic models were compared with real seismic records from the same stratigraphic setting (Chapters 2 and 7) or a similar situation (Chapters 5 and 6).
IMPEDANCE DISTRIBUTION
A crucial step in the construction of an outcrop-based seismic model is the conversion of the geologic cross-section into an impedance model showing the distribution of velocity and density (Part II). This can be done by directly measuring the acoustic properties of the rocks or by defining another variable, e.g. gamma-ray or resistivity, that follows the variation of impedance and can serve as a proxy of impedance. Chapter 8 shows how seismic models based on these measurements can be compared with seismic models based on sonic and density logs from boreholes. These comparisons will help to improve methods of converting outcrop data into realistic seismic models.
Erosional topography may be another possible proxy for impedance that is relatively easy to measure in outcrop. The underlying assumption is that resistance against erosion, "erosive hardness", is closely related to impedance, "acoustic hardness". In Chapter 9, photogrammetric techniques were used to establish the relationship between topography and impedance in the Vercors.
CONCLUSIONS
The lithologic detail modeled in the case studies lead to some striking results, particularly the discovery of pseudo-unconformities. Pseudo-unconformities are unconformities in seismics, but correspond to rapid changes of dip and facies in outcrop. In many case studies, lateral amplitude variations did not result from lateral variations in impedance contrasts, but from changes in depositional dip along carbonate platform slopes or from variable bed thickness.
Proxies of velocity, which can be more easily measured than velocity itself, include porosity (if over 10-15%), clay content (if over 10-15%), and bulk density (if both porosity and clay content are less than 10-15%). Another proxy of impedance is erosional relief, expressed as the slope angle.
The URL provides a link to the full report. The PDF contains the cover and Chapter 17 only.
Full reference:
Stafleu, J., van der Meulen, M.J., Gunnink, J.L., Maljers, D., Hummelman, J., Busschers, F.S., Schokker, J., Vernes, R.W., Doornenbal, H., den Dulk, M., and ten Veen, J.H., 2019. Systematic 3D subsurface mapping in the Netherlands; Chapter 17 in 2019 Synopsis of Current Three-Dimensional Geological Mapping and Modelling in Geological Survey Organizations, K.E. MacCormack, R.C. Berg, H.A.J. Russell, H. Kessler and L.H. Thorleifson (ed.), Alberta Energy Regulator / Alberta Geological Survey, AER/AGS Special Report 112, p. 179-190.
Modelling of surface and shallow subsurface data is getting more and more advanced and is demonstrated mostly for onshore (hydro)geological applications. Three-dimensional (3D) modelling techniques are used increasingly, and now include voxel modelling that often employs stochastic or probabilistic methods to assess model uncertainty. This paper presents an adapted methodological workflow for the 3D modelling of offshore sand deposits and aims at demonstrating the improvement of the estimations of lithological properties after incorporation of more geological layers in the modelling process. Importantly, this process is driven by new geological insight from the combined interpretation of seismic and borehole data. Applying 3D modelling techniques is challenging given that offshore environments may be heavily reworked through time, often leading to thin and discontinuous deposits. Since voxel and stochastic modelling allow in-depth analyses of a multitude of properties (and their associated uncertainties) that define a lithological layer, they are ideal for use in an aggregate resource exploitation context. The voxel model is now the backbone of a decision support system for long-term sand extraction on the Belgian Continental Shelf.
Human-induced groundwater level lowering in the Holocene coastal-deltaic plain of the Netherlands causes oxidation of peat organic matter, resulting in land subsidence and carbon dioxide (CO2) emissions. Here, a three-dimensional (3D) analysis of the distribution of the remaining peat organic matter is presented, to quantify the potential of this area to further subsidence and CO2 emissions by oxidation. Hereto, we established relations between dry mass ratios of organic matter and sediment in peat formed in different environmental settings. This was combined with a high-resolution 3D geological model of the subsurface of the Netherlands to map the proportions of organic matter, clastic sediment and void space in peat.
The 3D model indicates that c. 15 km3 of Holocene peat is embedded in the coastal-deltaic plain subsurface, of which c. 1.5 km3 consists of organic matter, 0.4 km3 of sediment, and 13.1 km3 of void space. During future human-induced oxidation, this peat has a volumetric loss potential of 14.6 km3, responsible for locally 0.4–6.0 m of subsidence, and a CO2 emission of 2.0 Gton.
The 3D modelling revealed that the amount of peat organic matter varies considerably between regions. Especially the subsurface of urban areas overlying back-barrier peat were identified as hot-spots accommodating the highest quantities of peat organic matter. The peat in agricultural areas contains less organic matter but is more prone to oxidation than peat underlying urban areas, because in the latter settings anthropogenic brought-up soil restricts oxidation. Future mitigation strategies should therefore focus on restricting peat oxidation in the agricultural areas of the coastal-deltaic plain.
Subsidence in the Holland coastal plain of the Netherlands was reconstructed from the vertical displacement of Holocene peat layers below their reference groundwater levels at the time of peat formation. This quantifies the part of subsidence that is due to compression processes and allows specification of the current state of peat compression in a map. 14C-dating of peat layers found intercalated in the Holocene sequence were used in the reconstruction. This dataset was combined with results from a recent coastal-deltaic plain wide three-dimensional (3D) interpolation of reference palaeo-groundwater levels, at which the intercalated peats are thought to have formed before they were buried, compressed, and vertically displaced. Empiric relations between reconstructed displacement and the thickness of overburden were determined and deployed in a national 3D geological subsurface model to establish a subsidence map with continuous cover of the coastal plain. The resulting maps show compressed peat layers under urbanized areas with 1 to 8 m of natural and anthropogenic overburden have subsided 1 to 5 m below the original level of formation. In the agricultural area of the coastal plain, where overburden is merely decimetres thick, consisting of fluvial flood- and sea-ingression deposits, peat generally experienced less than 1 m subsidence. The reference-level reconstruction method is deployable over large coastal plain areas to reconstruct subsidence caused by postdepositional vertical displacement of intercalated peat layers. It could therefore serve as an alternative approach for methods based on soil mechanics, which require input often not available for coastal plains on regional scales.
The urbanised peat-rich coastal-deltaic plain of the Netherlands is severely subsiding due to human-induced phreatic groundwater level lowering, as this causes peat layers to compress and oxidise. To determine the potential susceptibility of this area to future subsidence by peat compression and oxidation, the effects of lowering present-day phreatic groundwater levels were quantitatively evaluated using a subsidence model. Input were a 3D geological subsurface voxel-model, modelled phreatic groundwater levels, and functions for peat compression and oxidation. Phreatic groundwater levels were lowered by 0.25 and 0.5 m, and the resulting peat compression and oxidation over periods of 15 and 30 years were determined. The model area comprised the major cities Amsterdam and Rotterdam, and their surrounding agricultural lands. The results revealed that for these scenarios agricultural areas may subside between 0.3 and 0.8 m; potential subsidence in Amsterdam and Rotterdam is considerably lower, less than 0.4 m. This is due to the presence of several metres thick anthropogenic brought-up soils overlying the peat below the urban areas, which has already compressed the peat to a depth below groundwater level, and thus minimises further compression and oxidation. In agricultural areas peat is often situated near the surface, and is therefore highly compressible and prone to oxidation. The averaged subsidence rates for the scenarios range between 7 and 13 mm a −1 , which is corresponds to present-day rates of subsidence in the peat areas of the Netherlands. These results contrast with the trend of coastal-deltaic subsidence in other deltas, with cities subsiding faster than agricultural areas. This difference is explained by the driver of subsidence: in other deltas, subsidence of urban areas is mainly due to deep aquifer extraction, whereas in the Netherlands subsidence is due to phreatic groundwater level lowering.
The shallow subsurface of Groningen, the Netherlands, is heterogeneous due to its formation in a Holocene tidal coastal setting on a periglacially and glacially inherited landscape with strong lateral variation in subsurface architecture. Soft sediments with low, small-strain shear wave velocities (V S30 around 200 m s −1) are known to amplify earthquake motions. Knowledge of the architecture and properties of the subsurface and the combined effect on the propagation of earthquake waves is imperative for the prediction of geohazards of ground shaking and liquefaction at the surface. In order to provide information for the seismic hazard and risk analysis, two geological models were constructed. The first is the 'Geological model for Site response in Groningen' (GSG model) and is based on the detailed 3D GeoTOP voxel model containing lithostratigraphy and lithoclass attributes. The GeoTOP model was combined with information from boreholes, cone penetration tests, regional digital geological and geohydrological models to cover the full range from the surface down to the base of the North Sea Supergroup (base Paleogene) at ∼800 m depth. The GSG model consists of a microzonation based on geology and a stack of soil stratigraphy for each of the 140,000 grid cells (100 m × 100 m) to which properties (V S and parameters relevant for nonlinear soil behaviour) were assigned. The GSG model serves as input to the site response calculations that feed into the Ground Motion Model. The second model is the 'Geological model for Liquefaction sensitivity in Groningen' (GLG). Generally, loosely packed sands might be susceptible to liquefaction upon earthquake shaking. In order to delineate zones of loosely packed sand in the first 40 m below the surface, GeoTOP was combined with relative densities inferred from a large cone penetration test database. The marine Naaldwijk and Eem Formations have the highest proportion of loosely packed sand (31% and 38%, respectively) and thus are considered to be the most vulnerable to liquefaction; other units contain 5–17% loosely packed sand. The GLG model serves as one of the inputs for further research on the liquefaction potential in Groningen, such as the development of region-specific magnitude scaling factors (MSF) and depth–stress reduction relationships (r d).
A regional shear-wave velocity (VS) model has been developed for the Groningen gas field in the Netherlands as the basis for seismic microzonation of an area of more than 1000 km2. The VS model, extending to a depth of almost 1 km, is an essential input to the modelling of hazard and risk due to induced earthquakes in the region. The detailed VS profiles are constructed from a novel combination of three data sets covering different, partially overlapping depth ranges. The uppermost 50 m of the VS profiles are obtained from a high-resolution geological model with representative VS values assigned to the sediments. Field measurements of VS were used to derive representative VS values for the different types of sediments. The profiles from 50 to 120 m are obtained from inversion of surface waves recorded (as noise) during deep seismic reflection profiling of the gas reservoir. The deepest part of the profiles is obtained from sonic logging and VP–VS relationships based on measurements in deep boreholes. Criteria were established for the splicing of the three portions to generate continuous models over the entire depth range for use in site response calculations, for which an elastic half-space is assumed to exist below a clear stratigraphic boundary and impedance contrast encountered at about 800 m depth. In order to facilitate fully probabilistic site response analyses, a scheme for the randomisation of the VS profiles is implemented.
discuss modelling progress. We compared the models in terms of both methodology and usability. All three models were produced by the Geological
Survey of the Netherlands. Aggregate is granular mineral material used in building and construction, and in this case consists of sand and gravel.
On each occasion ever-increasing computer power allowed us to model at a higher resolution and use more geological information to constrain
interpolations. The two oldest models, built in 2005 and 2007, were created specifically for aggregate resource assessments, the first as proof of
concept, the second for an online resource information system. The third model was derived from the ongoing multipurpose systematic 3D modelling
programme GeoTOP. We used a study area of 40 x 40 km located in the central Netherlands, which encompasses a section of the Rhine-Meuse delta
and adjacent glacial terrains to the north. Aggregate resource assessments rely on the extent to which the occurrence and grain size of sand and
gravel are resolved, and on proper representation of clay and peat layers (overburden and intercalations) that affect exploitability. Average model
properties (e.g. total aggregate content) are about the same in all three models, except for a difference resulting from converting older lithological
classifications to the current one. This difference illustrates that data selection and preparation are paramount, especially when dealing with quality
issues. Generally speaking the results of the aggregate resource assessments are consistent and satisfactory for all three models, provided that they
are judged at the appropriate scale. However, the assessments based on GeoTOP best approach the desired scale of use for the aggregates industry;
in that sense progress was significant and each model was a better fit for the purpose.
The modelling procedure involved a number of steps. The first step is a geological schematisation of the borehole descriptions into units that have uniform sediment characteristics, using lithostratigraphical, lithofacies and lithological criteria. During the second modelling step, 2D bounding surfaces are constructed. These surfaces represent the top and base of the lithostratigraphical units and are used to place each voxel (100 by 100 by 0.5 metres) in the model within the correct lithostratigraphical unit. The lithological units in the borehole descriptions are used to perform a final 3D stochastic interpolation of lithofacies, lithology (clay, sand, peat) and if applicable, sand grain-size class within each lithostratigraphical unit. After this step, a three-dimensional geological model is obtained. The use of stochastic techniques such as Sequential Gaussian Simulation and Sequential Indicator Simulation, allowed us to compute probabilities for lithostratigraphy, lithofacies and lithology for each voxel, providing a measure of model uncertainty.
The procedures described above resulted in the first fully 3D regional-scale lithofacies model of the shallow subsurface in the Netherlands. The model provides important new insights on spatial connectivity of sediment units of, for example, sandy Holocene tidal channel systems. Our results represent a major step forward towards a fully 3D voxel model of the Netherlands.
Full reference:
Stafleu, J., Maljers, D., Busschers, F.S., Gunnink, J.L. and Vernes, R.W., 2011. TNO – Geological Survey of the Netherlands: 3-D Geological Modeling of the Upper 500 to 1,000 Meters of the Dutch Subsurface. In: Berg, R.C., Mathers, S.J., Kessler, H. & Keefer, D.A. (eds.): Synopsis of Current Three-dimensional Geological Mapping and Modeling in Geological Survey Organizations, Illinois State Geological Survey Circular 578, 64-68.
Two-dimensional seismic modelling techniques were used to determine the seismic-response of this interesting situation. To perform this, two different lithological models were constructed based on outcrops of the Sella south face. The first model is that of a rapidly prograding platform with slow aggradation in the platform interior. The second model shows clinoforms toplapping against the topset beds of the platform interior.
P-wave velocities and bulk densities were assigned to the lithostratigraphical units in accordance with values from a similar study, involving the same formations. The vertical-incidence method was used to construct perfectly migrated time sections and depth sections of reflectivity. These were convolved with zero-phase Ricker wavelets of different peak frequencies to produce the final synthetic seismic sections.
Using conventional, low frequencies (e.g. 25 Hz), the seismic response of the two models is almost identical. The topset beds and the Schlern-Raibl contact appear as one event. In a real seismic survey, both sections would be interpreted as toplap of Schlern clinoforms against the Raibl Formation. At higher frequencies (75 Hz), however, differences are revealed. The angle of progradation in the progradation & aggradation model becomes visible, as opposed to the horizontal surface in the progradation & toplap model. Topset beds are resolved separate from the Raibl Formation, but still appear to form a single dipping pseudo-toplap surface.
Another modelling technique, simulating unmigrated sections, shows few differences between the two models even at high frequencies. In addition, the clinoforms are disturbed by the refraction of rays. This study demonstrates that, even under ideal acquisition and processing conditions, the seismic tool can introduce a pseudo-toplap. This implies that toplap in a seismic section is not necessarily toplap in outcrop.
We have built two regional geological models-covering over 1,000 km 2-that serve as input for the seismic hazard and risk analysis of the onshore gas field of the Groningen region, the Netherlands. The first model describes the liquefaction potential indicators and the second model contains the layer model and parameterization for site response analysis. Earlier published papers focused on the construction of the models. The emphasis of the current paper is on the parameterization of both models. The liquefaction potential indicator consists of the cumulative thickness of loosely, moderately and densely packed sand in the top 40 m. This parameter was derived from the cone resistance values in the database of ~ 5,700 Cone Penetration Test (CPT) soundings. The geological model for site response consists of vertical voxel stacks corresponding to the GeoTOP model and extended to ~ 800 m depth using scenarios. These voxel stacks serve as input for site response calculations which requires information about shear wave velocity, unit weight, overconsolidation ratio, plasticity index, undrained shear strength, median grain size and coefficient of uniformity. These parameters were either derived based on local data from Seismic CPTs, CPTs or grainsize analyses. The empirical relations from literature were modified to fit the local Groningen characteristics of the soil. The parameterization was derived for the combinations of stratigraphy and lithology that are present in the region. Although our approach to schematize geology and to parameterize the geological models was developed for the region of Groningen, the general approach can be applied to other regions.
To anticipate on future resource supplies and needs, long-term adaptive management strategies for the exploitation of geological resources are urgently needed (cf. EU's Maritime Policy, Marine Spatial Planning, Marine Strategy Framework Directive). These are based ideally on a geological knowledge base incorporating comprehensive knowledge on the distribution, composition and dynamics of geological resources.
Such a knowledge base is being built for the Belgian and southern Netherlands part of the North Sea (Belgian Science Policy 'TILES') with the aim of providing long-term predictions of geological resource quantity and quality (Van Lancker et al., 2017, for an overview). Therefore, the lithology or sediment composition of the subsurface needs quantification which is traditionally derived from borehole point data. First these data are classified into classes, e.g., following the Wentworth scale (i.e., clay, silt, fine, medium to coarse sand and gravel), but secondly their distribution needs interpolation. Since the data are prone to a high variability, both in space and in depth, it is important to constrain the lithological interpolation within the geological unit or stratigraphy the lithoclass belongs to. This information ideally comes from seismic line data. Combining both point and line data is most efficiently done in a voxel modelling approach of which 3D volume elements are the key component. Advantages are the suite of geostatistical interpolations that can incorporate various criteria, including expert knowledge, the unlimited information that can be added to the data cube in a later phase, and the easy calculation of resource volumes per geological unit or resource quality. Importantly, the geostatistical modelling allows for probabilistic instead of static mapping providing the user with a measure of uncertainty on the lithoclass that is interpolated.
TNO – Geological Survey of the Netherlands (TNO – GSN) defines digital geological models as estimates of both geometry and properties of the subsurface. In contrast to singular observations in boreholes and the projected information of traditional maps, models provide continuous representations of the subsurface built with all geological expertise available. The models are quantitative and user oriented, i.e., they are applicable for non-geologists in their own area of expertise. They are also stochastic in nature, which implies that model uncertainty can be quantified.
TNO – GSN systematically produces 3D models of the Netherlands. To date, we build and maintain two different types of nation-wide models: (1) layer-based models in which the subsurface is represented as a series of tops and bases of geological, hydrogeological units and (2) voxel models in which the subsurface is subdivided in a regular grid of voxels attributed with a number of geological properties. Layer-based models of the shallow subsurface include the national geological framework model DGM (Gunnink et al. 2013) and the geohydrological model REGIS II (Vernes and Van Doorn 2005). A third layer-based model is DGM-deep with Carboniferous to Neogene seismostratigraphical units up to a depth of 7 km. The two main voxel models are the aggregate resources model (Maljers et al. 2015) and the multi-purpose GeoTOP model (Stafleu et al. 2011).
Our models are disseminated free-of-charge via the DINO-web portal (www.dinoloket.nl/en/subsurface-models) in a number of ways, including an on-line map viewer with the option to create virtual boreholes and cross sections through the models, and as a series of downloadable GIS products. A freely downloadable Subsurface-Viewer® was added to the portal, allowing users to download and visualize the layer-based models as well as GeoTOP on their desktop computers.
This extended abstract explores the three main models of the shallow subsurface, with an emphasis on the GeoTOP voxel model, discusses how we are currently integrating the layer-based models of DGM and GeoTOP and gives some examples of applications.
that each correspond with a particular application domain. Rather than the models themselves, we discuss
the meaning and implementation of working systematically, focusing on quality assurance and model
versioning and maintenance. Our aims for quality assurance are to put a system in place that arranges for
independent and documented checks of all model output, according to well-defined quality standards,
finding a balance between rigorousness on the one hand, and user demands to deliver information faster
on the other. Regarding model versioning and maintenance, we aim to significantly shorten our release
cycles, publishing models after making specific improvements rather than full updates. These two aspects
of working systematically are particularly important in helping us to be accountable in terms of the value
and quality of our modeling work, which in its turn is vital to the continuity of our Survey.
The GeoTOP model was used to perform a quantitative 3D spatial trend analysis on channel belt lithology, sand grain-size and architectural characteristics in the Holocene Rhine-Meuse delta. An analysis of the coarse-sand and fine-sand fractions shows clear spatial trends that relate to downstream changes in gradient, reworking of older sediments and tidal influence. Channel deposit proportions show an almost linear downstream decrease with an average value of about 0.5% per km. The analysis results can be used as input parameters for groundwater flow modeling studies in areas or at depths where this type of information is not available.
Modeling typically starts with a geological framework and this is then further refined to include user-specific parameters. In geohydrological applications, the geological framework model is used to distinguish geological units that act either as aquifer or as aquitard and to further parameterize these units with hydrological parameters, like porosity and hydraulic conductivity.
This extended abstract describes the parameterization for geohydrological applications of both the layer-based model REGIS II and the voxel model GeoTOP. The main emphasis is on building spatially distributed models of hydraulic conductivity, which are consistent with the geological model and can be used in groundwater flow modeling.
Hydraulic conductivity is a key parameter in geohydrological modeling. Direct measurements of hydraulic conductivity at the model-scale are often not available. Instead, hydraulic conductivity measurements are derived from pump-tests (which are costly and often multi-interpretable), from empirically derived relationships with grain-size distributions or by measuring hydraulic conductivity of small volumes of sediments that are extracted from boreholes. This results in the concept of “the missing scale” (Tran, 1996), and upscaling is necessary to derive meaningful parameters at the model-scale.
"
In contrast to the detailed knowledge of the 3D architecture of the Rhine-Meuse delta, little is known of the architecture of the Late Jurassic-Early Cretaceous deltaic system in the West Netherlands Basin at about 2000 m depth (Fig. 2). After having been explored for hydrocarbons during the last century, that basin is now receiving more and more attention for geothermal energy. As for hydrocarbon production, a key element for geothermal energy is an aquifer/reservoir with optimal connectivity.
Related to the topic of connectivity of fluvial channel deposits, we have started a pilot-study to test the applicability of the wealth of information contained in the detailed 3D model of the Rhine-Meuse delta for a better understanding of the distribution of fluvial bodies in general and in the Late Jurassic-Early Cretaceous deltaic system specifically.
(2) Modelling of 3D Rhine-Meuse channel belts - The GeoTOP model of the Rhine-Meuse delta was constructed using ~225.000 borehole descriptions from the DINO and Utrecht University databases. The location of the channel belts was derived from detailed maps published by the Geological Survey and the Utrecht University (Berendsen and Stouthamer, 2001). Newly developed software was used to determine the top and base of the belts within the boreholes. Finally, the use of stochastic interpolation techniques allowed us to predict lithology and sand grain-size for each voxel in the model.
(3) 3D lithology and grain-size trends - A preliminary 3D analysis of a single channel belt in the western part of the Rhine-Meuse delta (Figure 1) shows a clear downstream increase in percentages of fine-grained sand and a decrease in percentages of the coarser fractions. This observation, which illustrates the potential of the GeoTOP model to identify grain-size trends in sandy lowland rivers, initiated a full scale analysis of the entire delta in 3D. At ICFS10 we will present this delta-scale analysis and discuss the results in relation to other field- and model studies.
"
management, land subsidence, natural resources and infrastructural issues."
- GeoTOP-model covering the uppermost 30 meters;
- Digital Geological Model - shallow for the shallow sub surface to about 500 meters;
- Digital Geological Model - deep covering the deep subsurface to about 5000 meters.
These models in turn form the basis for the development of property models, like for example the national hydrogeological model REGIS II. All of these models are based on data from DINO, the central database for geoscientific data on the shallow and deep Dutch subsurface. See www.dinoloket.nl for more information.""
The TILES consortium has developed a 3D sand-resource model of the Quaternary deposits of the Belgian and Netherlands part of the North Sea to support marine spatial planning and exploration (TILES - Transnational and Integrated Long-term Marine Exploitation Strategies).
To continue reading, please refer to the PDF.
Voor een duurzaam gebruik en beheer van de ondergrondse ruimte is kennis en informatie over opbouw en eigenschappen van de ondergrond van groot belang. TNO-Geologische Dienst Nederland levert deze informatie in de vorm van driedimensionale modellen van de Nederlandse ondergrond.
In een live demo van DINOloket zullen we drie van de modellen de revue laten passeren: het diepe geologische model in het olie-en gasdomein (tot 4 à 5000 m diepte), het ondiepe ondergrondmodel in het grondwaterbereik (tot circa 500 m diep) en het meest gedetailleerde, ondiepe model GeoTOP (tot 50 m).
Vervolgens gaan we dieper in op het model GeoTOP, waarin de Nederlandse ondergrond in miljoenen voxels (blokken) van 100 bij 100 bij 0,5 m onderverdeeld is. We zullen zien hoe voor elk van deze voxels op basis van honderdduizenden boorbeschrijvingen en met behulp van complexe interpolatie-technieken geschat wordt tot welke stratigrafische eenheid de voxel behoort en wat de meest representatieve lithoklasse (veen, klei, zand) van de voxel is. Tot slot zullen we een aantal verrassende praktijktoepassingen van het model bekijken.
In 2006, TNO-Geological Survey of the Netherlands initiated the GeoTOP modelling program, aimed at constructing a detailed, national 3D geological voxel model of the shallow subsurface. We recently extended the model towards the southeastern part of the country, reaching a coverage of 28,605 km 2 (about 70% of the total land surface area; Fig. 1). GeoTOP schematizes the shallow subsurface in millions of voxels of 100 x 100 x 0.5 m up to a depth of 50 m below MSL. Each voxel contains multiple properties that describe the geometry of stratigraphical units (layers), the spatial variation of lithology and sand grain-size within these units as well as measures of model uncertainty. The addition of physical properties to the voxels enables the deployment of the model for a wide range of applications that benefit society.
Modelling is carried out in twelve model areas using a national database containing ~580,000 coded borehole descriptions and a context of geological maps created over the last few decades. The six existing model areas in the SouthWest , West and North of the country are characterized by a thick Holocene coastal wedge that is underlain by a stack of Pleistocene (sandy) units. The Holocene sequence includes thick occurrences of peat, which makes GeoTOP the de facto standard in predictive land subsidence studies. The model is also used in the estimation of ground motion acceleration caused by human-induced earthquakes in the Groningen gas field. The Rhine-Meuse delta in the central Netherlands is characterized by a complex of fluvial channel belt systems, which can serve as an analogue for hydrocarbon reservoir studies.
In contrast to these model areas, the newly added model area in the southeast is characterized by Pleistocene deposits, dissected by faults bounding the Roer Valley Graben. Syn-sedimentary faulting resulted in a complex pattern of dipping strata. Building a voxel model that takes these tectonic features into account appeared to be to be a challenging task. The addition of hydraulic conductivity to the voxels makes the model applicable in groundwater flow studies in the southeastern Netherlands.
In the next couple of years, we will upgrade some of the older model areas in order to meet the requirements of the Key Register of the Subsurface (BRO) of which GeoTOP will become a part.
TNO-Geological Survey of the Netherlands (GSN) develops and maintains a suite of four subsurface models. The GeoTOP voxel model is our most detailed model with a coverage of ~70% of the Netherlands. This detail is made possible by using all borehole data available meeting our quality criteria and by inserting as much geological knowledge as possible for example by reusing existing maps and other publications as well as newly developed geological conceptual models.
Using all available borehole data however asks for automated interpretation procedures, because of the vast amount of data available (~500.000 coded borehole descriptions stored in our national database). We have created an interpretation toolbox programmed in Python to ensure a standard method. With this toolbox we are able to stratigraphically interpret lithological intervals in boreholes using their position in the XY and Z-direction, the assumed stratigraphic superposition of geological units and the lithological properties of the described interval. By doing so we hugely enlarge our dataset of stratigraphically interpreted boreholes, and we eliminate subjectivity within the stratigraphic interpretation.
Cone penetration test data (CPT data) is commonly acquired in the planning stage of infrastructural works. Along line elements such as railroads and motorways a spacing of ~25 m is typical. In the Netherlands, this kind of data therefore vastly outnumbers the available boreholes. Up till now, CPT data was not used for the modelling of subsurface geometries and properties. We have recently demonstrated that by using Artificial Neural Networks we are able to interpret CPT data into facies units using a combination of cone resistance, friction ratio and depth. This interpretation together with the automated interpretation of boreholes forms the input data for a detailed subsurface model. In addition, the lithological composition within the model is determined by using a simple lithological interpretation of the same CPT data by using the so-called three type-rule in combination with lithological borehole information.
By using automated procedures we can use more data than ever before. This enables us to make more detailed subsurface models. However using more data of which the interpretation has not been manually checked asks for (partly automated) quality procedures to ensure overall quality.
Geological databases resulting from the merging of various data sources and time periods jeopardize harmonization of data products. Data standardization is already common practice and a first step in avoiding semantic overlap. European marine data-management infrastructures provide such standards, e.g., Geo-Seas (http://www.geo-seas.eu/) for geological data and SeaDataNet (https://www.seadatanet.org/) for marine metadata in general. In addition, metadata quality control is important, though data uncertainty is seldom quantified and yet to be used in modeling. Preliminary uncertainty analyses were worked out to provide an extra dimension to the cross-border 3D voxel models of the geological subsurface of the Belgian and southern Netherlands part of the North Sea (http://odnature.naturalsciences.be/tiles/). Starting from simple quality flagging in geological databases and model-uncertainty calculations (probability and entropy) in the 3D modelling, data uncertainty (e.g., related to qualities in positioning, sampling and vintage) is now quantified. Combining all uncertainties remains a challenge, as is communicating their importance in decision making. A demonstration will be given on the status of the uncertainty analyses and on the way these are incorporated into a newly developed decision support tool allowing interactive querying of the 3D voxel model, now comprising geological parameters as well as entropy, probability and data-uncertainty attributes (Figure 1).
Figure 1. Interactive querying of the 3D geological voxel model in the newly developed 2D Decision Support System (DSS).
Sand-stock assessments should include the quality of the resource. 3D pixel (voxel) models incorporate multiple properties and allow in-depth analyses of their interrelationships. Because of the structured geometry, voxels capture the 3D-spatial heterogeneity within a resource layer better than maps.
Geological voxel models subdivide the subsurface in a regular grid of rectangular blocks ('voxels', 'tiles' or '3D cells') in a Cartesian coordinate system. Each voxel contains multiple properties that describe the geometry of stratigraphical units and the spatial variation of lithology within these units. Using voxel models, the architecture and internal heterogeneity of stratigraphical units in the subsurface can be modelled in great detail. By assigning physical properties to the voxels we are able to turn the models into powerful instruments for a wide range of applications such as: groundwater management, risk assessments, the planning of infrastructural works and aggregate resource assessments. The underlying assumption is that the spatial variation of many subsurface properties, such as hydraulic conductivity and shear-wave velocity, strongly depends on the two main geological properties in the model: stratigraphy and lithology (Figure 1). Fig. 1: Putting geological voxel models to work by adding properties related to the application at hand. In this presentation, we will show the following real life applications of geological voxel models attributed with physical properties: 1) the Netherland's nationwide mapping programme GeoTOP, 2) a detailed model of the subsurface of Tokyo Lowland, Japan, and 3) a voxel model of the Belgian Continental Shelf. These models cover the upper 50 m of the subsurface. The GeoTOP model covers 23,325 km 2 (57%) of the surface area of the Netherlands. It is a multipurpose model that has already been used in a number of different applications. A recent application is the risk-assessment of damage caused by human induced earthquakes in the Groningen gas field (Figure 1). Another GeoTOP-application is the long-term prediction of land subsidence due to the oxidation and compression of peat layers. The model of the Tokyo Lowland was used to study the relation between the accumulated thickness of soft Holocene mud and the amount of damage caused by natural earthquakes. The Quaternary sands of the Belgian Continental Shelf are a resource for the construction industry and for the reinforcement of the Belgian coast. A voxel model based on shallow seismic profiles and a limited amount of boreholes was developed and subsequently complemented with an online query-tool. This tool enables the users to perform volume calculations, which are crucial for the management of raw materials in the marine environment. These case studies exemplify the value of geological voxel models in real life applications. Furthermore, they show that voxel models attributed with physical properties are deployable in a wide range of geological settings.
TNO-Geological Survey of the Netherlands (GSN) develops and maintains a suite of four subsurface models. These models each have their own historical background, use different data, different modelling techniques and serve different user groups. However, today more and more the call for integration of these subsurface models is heard. Therefore at GSN we are currently working on the integration of two of these models covering the shallow subsurface. The result is a single, integrated and multiresolution model that displays a great amount of detail in the upper tens of meters, but at the same time reaches, albeit with less detail, depths of several hundreds of meters. In doing so we eliminate differences between realizations of the same geological units. The integration of the shallow framework models appear to be a relatively straightforward step, mainly because they are constructed using comparable datasets (mainly boreholes) and the same modelling software, but is nevertheless time-consuming. The new integrated model will serve as the future carrier of our voxel models with detailed lithological information as well as our hydrogeological information on aquifers and aquitards. In recent years, progress has been made in the integration of our shallow and deep subsurface models in the context of H3O projects. These projects, carried out in close collaboration with our Belgian and German partners, deliver cross-border hydrogeological models. The main challenges in these projects are the harmonisation of the stratigraphic nomenclatures used on either side of the border, the integrated interpretation of data from boreholes as well as from seismic lines, the handling of faults, and the use of different software. In the Netherlands, due to the energy transition, geothermal energy receives more and more attention. At greater depths, this energy source often come from reservoir rocks that are already modelled in detail for hydrocarbon exploration. However, the recent shift of focus to more shallow reservoirs in the Netherlands has, to date, received little attention from the geomodelling community. The main reason is that these shallow reservoirs fall outside the depth ranges of the shallow models and lack detail in the deep models, because they were irrelevant for hydrocarbon exploitation (Figure 1). Although here only one example underpinning the needs for integration is mentioned, the increased use of the subsurface in providing essential resources and storage capacities (and the associated synergies) asks for better integration of subsurface models. Although we do not have all the answers to the way ahead yet, we look forward to discuss the possible approaches for model integration with the audience. Fig. 1: Example of a cross-section of a shallow subsurface model (upper panel) and a deep subsurface model (lower panel). Interest is currently focussed on the depth range of 600-1200 m below reference level, which is not modelled in the shallow model and has received little attention in the deep model.
The Geological Survey of the Netherlands maintains a portfolio of four national subsurface models. Layer-based models include the geological framework models DGM (Digital Geological Model) and DGM-deep, and the hydrogeological model REGIS-II. The distinction between deep and shallow modelling relates to both the application of the model and modelling methods. Shallow modelling, having evolved from traditional geologic mapping, is primarily based on the correlation of boreholes and covers depths that are relevant to geotechnical and groundwater studies (generally down to about 500 m below the surface). Deep modelling, originally targeted at hydrocarbon resources, primarily uses seismic data down to about 5 km below the surface. Voxel (i.e. 3D raster) models of the upper tens of meters of the subsurface include GeoTOP, a high-resolution model that is in the process of being built and covers about half of the country, and NL3D, a lower-resolution model that already has national coverage. Developments in Dutch geomodelling after the previous status update (Wiesbaden, 2016) include the following highlights: (1) GeoTOP now includes all coastal provinces and the Rhine-Meuse delta, serving the areas that are most vulnerable to adverse ground conditions and flooding. Research preparing for geochemical and hydraulic parametrisation of GeoTOP is well underway, to be eventually followed by geotechnical parametrisation. (2) A new version of REGIS-II has been released, which is geometrically compatible with the last release of DGM (2014). (3) The layer modelling step of GeoTOP has been integrated with that of DGM, in order to enhance geometrical consistency. (4) Following on a first project that started in 2014, two more transboundary hydrogeological models are being constructed together with counterpart organisations in Belgium and Germany. The results will present water managers in the border areas with better (seamless) information, and the exchange of knowledge between project partners boosted our collective understanding of the regional (hydro)strati-graphy. The results of the individual projects will be fed back in the DGM, REGIS-II and DGM-deep programmes. Beyond technical-scientific progress, pending developments in Dutch geomodelling primary relate to a new law on subsurface information. Since 2010, our Survey has been preparing for a transformation of our databases to a 'key register for the subsurface' (further referred by its Dutch acronym BRO). A key register is legally defined register containing high-quality data that the government is obliged to use for its public tasks, existing ones containing personally identifiable information; identification and ownership of real estate, companies and vehicles; real-estate value; income, employment relations and social-security benefits; addresses and buildings; and base topography. The BRO recognises the government's reliance on subsurface data and information for a number important planning and permitting procedures, e.g., for land use planning; exploration and production of hydrocarbons, minerals and geothermal heat; storage of CO2 and natural gas; and groundwater management. Beyond that, it is expected to help reduce the considerable societal risks and costs associated with adverse ground conditions in public works, especially infrastructure projects. The BRO law is now in a process of stepwise implementation, i.e., datatype by datatype, which will take several years. For systematic geomodelling, the BRO law is crucial: it is the only way in which we can substantially increase the inflow of data to our survey. In this way, we expect the law to be instrumental in achieving higher model resolutions at lower uncertainties.
Already for 1000 years, the Netherlands has seen steady human-induced subsidence. There is no reason why subsidence would not continue the coming decades, as the root causes are still active. Subsidence causes an increased flood risk, which has to be compensated by continuous investments in water management, and it also may cause damage to infrastructure and constructions.
The resulting costs are accumulating to billions of euros per year, and these costs will rise in the future, as the exposure and vulnerability of society to land subsidence increases. Predictive maps of land subsidence are used in policy to mitigate subsidence and its effects, or are used in efforts to become more adaptive.
The results encouraged us to start further improvement of national subsidence modelling, in terms of the capability to deal with large datasets, and the subsidence model code.
The BCS until recently regarded as one of the most well-studied areas of the North Sea has more to show than originally thought. Buried valleys, deltaic units, tidal channels, and erosional unconformities provide a challenging environment for 3D modelling. Within the frame of the Belspo Brain-be project TILES (Transnational and Integrated Long-term Marine Exploitation Strategies), a
3D voxel model of the subsurface of the North Sea will be created. The borehole and 2D seismic database of Belgium and Holland were combined to create the geological scheme of the area, unified borehole lithological descriptions and 2d bounding surfaces determining lithostratigraphical units. The previously mentioned data were interpolated using ISATIS to create the 3D voxel model.
Each voxel is describing either a unique value of a lithological feature (fine sand, gravel, etc.) or the occurrence probability of it. Results from the probability voxel model, showed a more detailed distribution of Pleistocene to Holocene stratigrafical units, outlined the deltaic fan of the Schelde River and aiso provided a preliminary model that can be used to determine resources volumes and their sustainable exploitation and in planning coastal zone and offshore area development.
The need for marine space grows bigger by the year. Dredging, wind farms, aggregate extraction and many other activities take up more space than ever before. As a result, the need for an accurate model that describes the properties of the areas in use is a priority. To address this need a 3D voxel model of the subsurface of the Belgian part of the North Sea has been created in the scope of the Belgian Science Policy project TILES ('Transnational and Integrated Long-term Marine Exploitation Strategies'). Since borehole data in the marine environment are a costly endeavour and therefore relatively scarce, seismic data have been incorporated in order to improve the data coverage. Lithostratigraphic units have been defined and lithoclasses are attributed to the voxels using a stochastic interpolation. As a result each voxel contains a unique value of one of 7 lithological classes (spanning in grain size from clay to gravel) in association with the geological layer it belongs to. In addition other forms of interpolation like sequential indicator simulation have allowed us to calculate the probability occurrence of each lithoclass, thus providing additional info from which the uncertainty of the model can be derived. The resulting 3D voxel model gives a detailed image of the distribution of different sediment types and provides valuable insight on the different geological settings. The voxel model also allows to estimate resource volumes (e.g. the availability of particular sand classes), enabling a more targeted exploitation. The primary information of the model is related to geology, but the model can additionally host any type of information.
Geological mapping of Holocene deposits of coastal plains, such as that of The Netherlands can reach high resolution (dense population, diverse applied usage) and good time control (14C dating, archaeology). The next step is then to create time sliced reconstructions for stages in the Holocene, peeling of the subrecent and exposing past relief situation. This includes winding back the history of sea-level rise and delta progradation etc. etc. So far, this has mainly be done as 2D series of landscape maps, or as sea-level curve age-depth plots.
In the last decade, academic and applied projects at Utrecht University, TNO Geological Survey of The Netherlands and Deltares have developed palaeoDEMs for the Dutch low lands, that are a third main way of showing coastal plain evolution. Importantly, we produce two types of palaeoDEMs: (1) geological surface mapping using deposit contacts from borehole descriptions (and scripted 3D processing techniques – e.g. Van der Meulen et al. 2013) and (2) palaeogroundwater surfaces, using sea-level and inland water-level index-points (and 3D kriging interpolations – e.g. Koster et al. 2016). The applications for the combined palaeoDEMs range from relative sea-level rise mapping and assessment of variation in rate of GIA across the coastal plain, to quantification of soft soil deformation, to analysis of pre-embankment extreme flood levels.
Koster, K., Stafleu, J., & Cohen, K.M. (2016). Generic 3D interpolation of Holocene base-level rise and provision of accommodation space, developed for the Netherlands coastal plain and infilled palaeovalleys. Basin Research. DOI 10.1111/bre.12202
Van der Meulen, M.J., Doornenbal, J.C., Gunnink, J.L., Stafleu, J., Schokker, J., Vernes, R.W., Van Geer, F.C., Van Gessel, S.F., Van Heteren, S., Van Leeuwen, R.J.W. & Bakker, M.A.J. (2013). 3D geology in a 2D country: perspectives for geological surveying in the Netherlands. Netherlands Journal of Geosciences, 92, 217-241. DOI 10.1017/S0016774600000184
For long-term predictions of geological resource quantity and quality, a voxel model was built for the subsurface of the Belgian part of the North Sea (Belgian Science Policy ‘TILES’). The 3D voxels contain lithostratigraphic information over the entire data volume (up to -70 m), but also sediment characteristics and a suite of sediment-dynamic parameters in the upper voxel (i.e., the seabed). Derivative data products include probability maps of sediment type and resource-suitability maps that reflect a combination of user-specific criteria.
From stakeholder consultation, it became clear that the model has numerous potential applications, provided that resolution requirements from very small-scale to large-scale can be met (e.g., assessing local aggregate quality, but also decision-making on long-term resource use). To accommodate these varying needs, and also to enhance computational speed, voxel models were post-processed to vary the size of the voxels dependent on user demand. As such, data density, but also geological heterogeneity can steer the voxel size.
Uncertainty is parameterised to generate data products with confidence limits. Uniquely, the quality of each of the data fields in the databases is quantified in order to be propagated in the voxel model. Additionally, interpolation-related uncertainty, as well as uncertainty in the mapping of the lithological class and stratigraphic uncertainty (i.e. the geological layer to which a so-called lithoclass belongs) is incorporated. Visualisation of these uncertainties is highly challenging, and is addressed through variation in the sizes of the voxels or through transparency.
An ample spectrum of benefits exists for habitat mapping. Firstly, the geology of the shallow subsurface is accounted for, being highly relevant in predicting and constraining habitat change in long-term projections of resource use. The approach is also pertinent to assist present-day seabed mapping. Uncertainty mapping is critical to judge on the accuracy of seabed maps, and it assists in interpreting habitat changes within an envelope of natural variability, imposed by both the geological nature of the seabed and the highly dynamic sedimentary environment.
Mineral and geological resources are non-renewable on time scales relevant for decision makers. Once exhausted by humans, they are not replenished rapidly enough by nature, meaning that truly sustainable resource exploitation is not possible. Comprehensive knowledge on the distribution, composition and dynamics of geological resources and on the environmental impact of extraction is therefore critical. Anticipating on this, a geological knowledge base has been developed as a platform for resource management (Belspo Brain-be TILES, 2014-2018). Backbone of the knowledge base is a 3D voxel model (volume pixels) of the surface and subsurface of the Belgian and southern part of the North Sea. Standardized and harmonized databases have been created and a methodological workflow for the 3D modelling of offshore aggregates published. Data were added to the highest detail as to maximize their classification to any application (e.g., aggregate industry). Metadata were carefully added to estimate data-related uncertainty. The 3D geological models were further coupled to 4D numerical environmental impact models as to quantify environmental impact under various scenarios of exploitation. Furthermore, the voxels were filled with decadal sediment transport calculations allowing assessing seabed recovery estimations after extraction, an important asset in many environment-related European Directives. Data, models, and their uncertainties, are embedded in an end-user driven decision support system (DSS) that uniquely allows querying the full 3D resource volume, and integrating it with any third-party data. Visualization is an inherent part of the DSS, but to maximize impact towards a broader user community, a virtual reality application has been built too. From a management perspective, the DSS allows long-term resource predictions, balancing aggregate quality and quantity against various applications, and whilst minimizing environmental impact. Finally, a vision will be presented on how incorporating the geological knowledge base into a future national seabed mapping programme.
Sustainable management of marine geological resources starts with a deep understanding of their diverse qualities and quantities. To facilitate comprehension of these variables a 3D voxel model of the subsurface of the Belgian part of the North Sea has been created in the scope of the Belgian Science Policy project TILES ('Transnational and Integrated Long-term Marine Exploitation Strategies'). The construction of the model follows a standardised methodological approach that is versatile in the addition of data that comes from different sources. In this case the voxels are filled with geological data originating from boreholes and seismic lines. The resulting 3D voxel model gives a detailed image of the distribution of different sediment types and provides valuable insight on the different geological settings. The voxel model also allows estimating resource volumes (e.g. availability of particular sand classes), enabling a more targeted exploitation. The primary information of the model is related to geology, but the model can additionally host any type of information, e.g., relevant from an industry perspective. Web querying tools have been developed of which the functionalities were mainly driven by end-users. Furthermore, a virtual reality application was built projecting the 3D models in their true dimensions and allowing more comprehensive interaction with the viewer.
Geological voxel models subdivide the subsurface in a regular grid of rectangular 3D cells in a Cartesian coordinate system. Each voxel contains multiple properties that describe the geometry of stratigraphical units and the spatial variation of lithology within these units. The addition of physical properties to voxels enables the deployment of these models for a wide range of applications such as: groundwater management, risk assessments, the planning of infrastructural works and aggregate resource assessments. In this presentation, we show real life applications of the following three geological voxel models attributed with physical properties: 1) the Netherland's nationwide mapping programme GeoTOP, 2) a detailed model of the subsurface of Tokyo Lowland, Japan, and 3) a voxel model of the Belgian Continental Shelf. These models cover the upper 50 m of the subsurface. 1) A recent application of the GeoTOP model is the risk-assessment of damage caused by human induced earthquakes in the Groningen gas field. Another GeoTOP application is the long-term prediction of land subsidence due to the oxidation and compression of peat layers. 2) The model of the Tokyo Lowland was used to study the relation between the accumulated thickness of soft Holocene mud and the amount of damage caused by natural earthquakes. 3) The Quaternary sands of the Belgian Continental Shelf are a resource for the construction industry and for the reinforcement of the Belgian coast. A voxel model of this area was developed and subsequently complemented with an online query-tool. This tool enables users to perform volume calculations, which are crucial for the management of raw materials in the marine environment. These case studies exemplify the value of geological voxel models in real life applications. Furthermore, they show that voxel models attributed with physical properties are deployable in a wide range of geological settings.